IS Families/IS21 family
General
IS21 was discovered in plasmid R68 where it was subsequently observed to undergo a tandem duplication which greatly facilitated the insertion of the plasmid into the Pseudomonas host genome. This led to the formation of Hfr strains[1][2] and the subsequent demonstration that the Pseudomonas chromosome was genetically circular[3] (Fig.IS21.1).
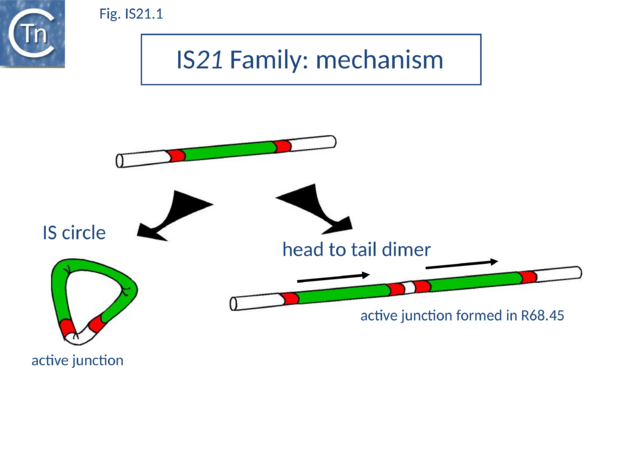
The conjugative plasmid R68 carries a single copy of IS21 and is inefficient in chromosome transfer [1]. An R68 derivative, R68.45, exhibiting a marked increase in transfer of chromosomal genes, was isolated [1] and proved to carry two directly repeated copies of IS21 separated by 3 bp[4] (Fig.IS21.1).
In addition to the distinct examples restricted to individual species, several individual ISs are themselves quite widely distributed. Isoforms of IS21, several of which are incomplete, have been found in RP4 (IS8[5]), in Shigella sonnei, and various clinical isolates of Escherichia coli, in the EHEC plasmid p0157[6] and in the Yersinia pestis virulence plasmid pCD1 (IS640[7]; IS21p). Another family member, IS100 is found in several Yersinia plasmids, including pCD1[8], flanking a high pathogenicity island in Y. pestis (as well as multiple copies in the genome) and Y. pseudotuberculosis[9], and in the uropathogenic E. coli J96[10].
The IS21 family is fairly homogeneous. Family members have lengths in the range 1850-2800bp and are therefore among the largest bacterial IS elements (TABLE Characteristics of IS families). They carry related terminal IRs whose lengths may vary between 11 (e.g., IS21) and 50 bp (e.g., IS5376) and generally terminate in the dinucleotide 5'-CA-3' (Fig.IS21.2; IS21.3 and .IS21.4).
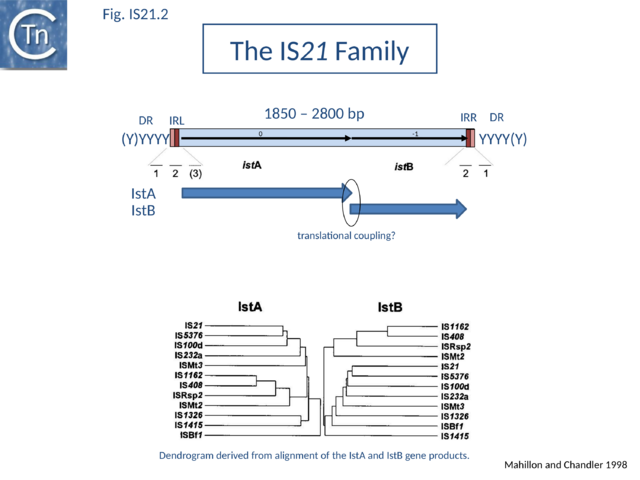
The IS21 family terminal IRs are complex. Several carry multiple repeated sequences at their ends which include part of the terminal IRs[11] (Fig.IS21.2) and which may represent Tpase binding sites. IS21 itself also carries a degenerate set of such sites (Berger B, 2000, La séquence d’insertion IS21: la famille de cette élément bactérien, sa spécificité d'insertion et son utilisation pour une méthode de linker insertion mutagenesis in vitro, Université de Lausanne undergraduate thesis) which were not initially identified. Like a number of IS families, the ends of the element terminate with the dinucleotide 5’-CA-3’ (Fig.IS21.4).
Insertion results in a direct target repeat of 4 or, more frequently, 5 bp while two members (eg., IS53 and IS408) may generate 8 bp.
IS21 family members encode two consecutive genes, along upstream frame designated istA the Tpase and a “helper” gene, and a shorter downstream frame, istB (Fig.IS21.2) which exhibits some similarity to the DnaA replication initiator protein due to the presence of an ATP binding motif, and often appears in BLAST searches of complete genomes.
Overall identities range from 10 to 59% for IstA and from 25 to 67% for IstB. For one member, IS53[12], a match of 45% identity of the potential istA frame with that of IS1326 can be obtained by the introduction of several frameshifts. An additional sequence, IS640[13] from Shigella sonnei, is a truncated iso-IS21. It carries an istA reading frame which is nearly identical to that of IS21 but is lacking istB and the right terminal IR. Many additional IS21 derivatives are appearing in the various bacterial genome sequences.
The istB frame may be located in a relative reading phase of -1 (e.g. IS21, IS5376) or +1 (e.g. IS232, IS1326) compared to istA. It can be slightly separated from istA (17 bp, IS408 - for which only a partial sequence lacking the right end is available) or overlap for one (IS21) or several base pairs (IS232, IS5376, IS1326), and is generally preceded by a potential ribosome binding site. In many members, the termination codon of istA and the initiation codon of istB overlap suggesting that IstB is produced by translational coupling (Fig.IS21.2).
The istA reading frame carries a motif related to the widespread integrase DDE motif but lacking the conserved K/R residue and a potential helix-turn-helix motif. Although there is no general conservation of the K/R residue expected seven residues downstream from the final E , there is good conservation of a basic amino acid at either position 7 or 8 (Berger B, 2000, La séquence d’insertion IS21: la famille de cette élément bactérien, sa spécificité d'insertion et son utilisation pour une méthode de linker insertion mutagenesis in vitro, Université de Lausanne undergraduate thesis). The istB frame carries a relatively well conserved potential NTP binding domain[14]. Sequence alignments of IstA and of IstB gives very similar relationships [11].
IS21
IS21 has been studied in some detail. The tandem duplication observed in the original R68.45[4] can be envisaged to occur by various routes. It is presumably favoured by the marked tendency of IS21 to insert in, or close to, an IS21 end[15]. It may also occur by unequal crossing over during replication of the donor plasmid or, as suggested for IS30, whose transposition mechanism shows some similarity with that of IS21 (see "IS30 family"), by dimerization of the donor plasmid and deletion of one intervening plasmid sequence located between the two IS copies (Fig.IS21.1). This type of mechanism has been demonstrated experimentally both for IS30[16] and for IS911[17]; IS3 family). The tandemly repeated copies of IS21 promote insertion of the entire plasmid in a transposition event involving the abutted terminal IRs. Integration results in loss of the 3 bp located between the two ends in the parental R68.45 plasmid.
The resulting replicon fusion thus has the appearance of a true cointegrate (i.e. with a single directly repeated copy of the IS at each junction) although transposition does not involve duplication of the insertion sequence during the insertion event.
Both IstA and IstB are required for integration[18] and production of a second IstA derivative (from an alternative translational initiation codon eight codons downstream of that of IstA itself) greatly increases the efficiency of replicon fusions [18] for reasons that are at present unknown Integration is optimal when the distance separating the two ends is 4 bp. It is efficient with a 2 or 3 base pair separation but inefficient with smaller or larger intervening sequences[19]. Like retroviruses, and several other transposable elements, IS21 terminates with a 5'-CA-3'. Mutation of the terminal A abolishes replicon fusion (Haas et al., 1996, Insertion sequence IS21: Related insertion sequence elements, transpositional mechanisms, and application to linker insertion mutagenesis, in Nakazawa T, Molecular biology of Pseudomonads).
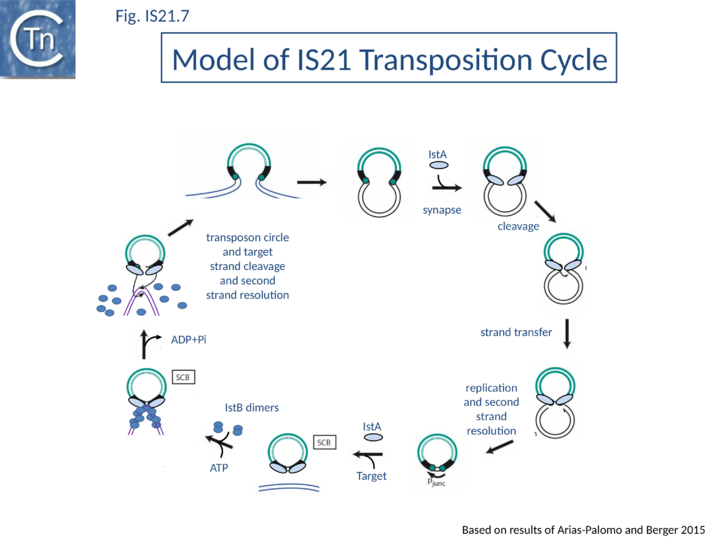
The duplication of IS21 in R68.45 not only creates an efficient integrative donor structure by providing two closely spaced and correctly oriented IRs, but generates a strong promoter (as measured with a galK reporter gene) in which a -35 box located in the IRR of the upstream element is combined with a -10 box located in the abutted IRL of the downstream copy. This directs high levels of production of the IS21 transposition proteins [4] in a similar manner to the junction promoter created by circularization of IS911 and IS2. The insertion of IS21 downstream of strong promoter results in the efficient expression of transposition proteins. Thus IS21 appears not to have adopted a mechanism which "protects" it against high levels of impinging transcription. It is presumably the necessity for a transposition substrate carrying two abutted ends and the low frequency of formation of such molecules which acts as a control on transposition activity. More recently, a low level of circular IS21 copies has been detected in preparations of IS21-carrying plasmid DNA[20] (S. Schmid and D. Haas, pers.comm.), raising the possibility that IS21, like at least some of the IS3 family members, may also transpose via a circular intermediate.
It has been demonstrated in vitro, using E. coli cell extracts enriched for IstA, that this protein is responsible for the 3' end cleavage of IS21[18]. A substrate carrying two abutted IS21 ends separated by 3 bp is cleaved specifically at both 3' ends in an IstA-dependent way [see [20]].
Current data are consistent with the notion that IS21 family members transpose using a two-step mechanism (Fig.IS21.7) by the formation of a reactive junction, similar to those formed in the copy-paste mechanism of IS3 and other families, in which two abutted IS21 ends are separated by several nucleotides. This is consistent with the marked tendency of IS21 to insert in, or close to an IS21 end. The reactive junction is subsequently integrated into the target DNA to give a DR of 4 to 8 bp. Integration is optimal when the distance separating the two ends in the junction is 4 bp. It is efficient with a 2- or 3-bp separation but inefficient with smaller or larger intervening sequences. IstA carries the DDE motif and is the Tpase. IstB is necessary for the target DNA capture and accurate insertion by IstA donor insertion by its cognate transposase [18].
Both IstA and IstB are required for efficient transposition while a product of alternative natural translation initiation within the Tpase gene (which removes the eight N-terminal amino acids) may facilitate integration to generate “cointegrates” and has been called a “cointegrase”[19].
Biochemical and structural data are now available concerning the role of IstB and IstA in IS21 transposition[21][22]. Several transposable elements use regulatory AAA+ ATPases for function. For bacteriophage Mu (MuB) and Tn7 (TnsC), these proteins are involved in the choice of target sites[23][24]. IS21, IstB also carries a conserved potential NTP binding domain (Fig.IS21.5) which shares sequence similarity with the DnaA protein and because of this, often interferes with automatic bacterial genome annotation pipelines.
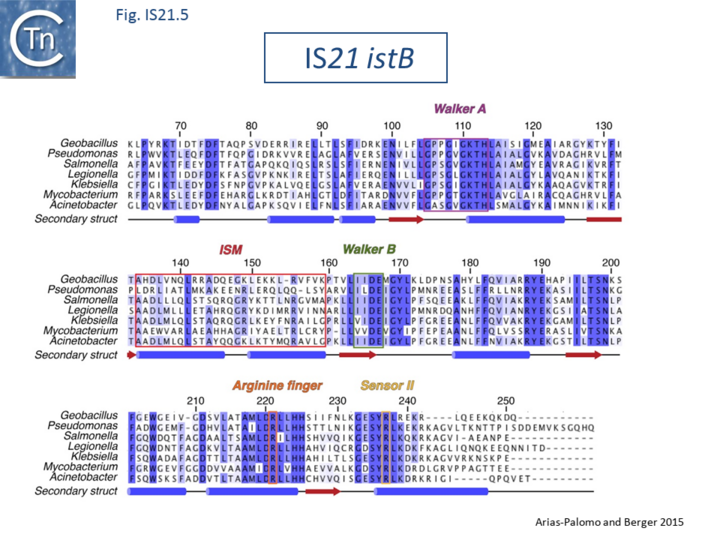
The X-ray crystallographic structure of IstB with its N-terminal domain removed including a bound inactive ADP-BeF3 ligand superposed well onto the AAA+ domain of DnaC (Fig.IS21.6). X-ray crystallography and cryo-electron microscopy showed that the full length IstB protein self-assembles into a helical decamer (a pentamer of dimers; a so-called clamshell-shape) forming a right-handed filament very similar to that of DnaC and MuB[25] (Fig.IS21.6). Multimerization is dependent on the ATP-co-ordinating residues. This filament was capable of grasping DNA between two pentamers generating a 180° bend and also to introduce positive supercoils in an ATP dependent manner presumably by wrapping the DNA. Binding appears to show no sequence-specificity.
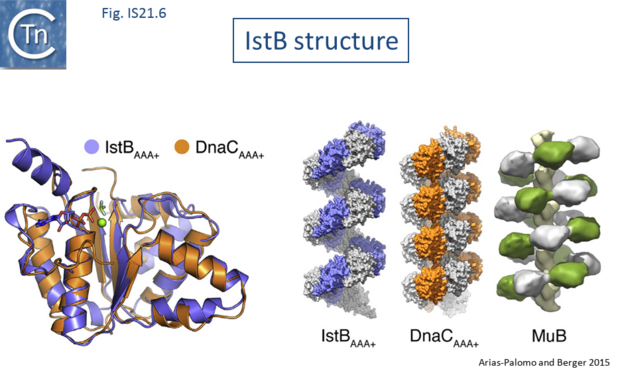
The transposase, IstA, was also shown to recognize the IstB DNA complex and to promote disassembly by stimulating ATP hydrolysis.
A model has been proposed in which IstB binding and subsequent DNA bending primes a selected insertion site for efficient transposition: an IstB decamer first associates with target DNA, and the complex then recruits IstA in the form of a transpososome or paired-end complex (PEC) including a pair of inverted IS21 repeats to generate a synaptic complex. Although it has been proposed that the PEC has precleared ends, this is not an essential requirement. The order of strand cleavage and the multiple stages of transpososome assembly must yet be defined. The inability of DNA to promote ATP turnover by IstB would impart sufficient stability and allow time for IstA recognition. IstA-stimulated ATP hydrolysis would lead to IstB dissociation to a clearing of the DNA, allowing the IstA access to the target DNA. In this model, IstB might be considered an AAA+-type co-chaperone[21] (Fig.IS21.7).
IS21 has been reported to show a preference for insertion close to the end of a second copy of the element located in the target plasmid[15]. This is a characteristic of many IS families whose members transpose via a circular dsDNA intermediate and presumably reflects their ability to form a synaptic complex between an IR carried by the intact IS and a second IR in the target molecule (see Fig.IS3.11).
Probing Mechanism
The IS21 family transposition mechanism has been investigated using biochemical and structural approaches [22] with the thermophilic Geobacillus stearothermophilus IS5376 [26] as a model.
A classical cell-free system originally developed for phage Mu and retroviral integrases [27][28] was set up. This uses double-stranded DNA fragments carrying the terminal IS5376 55bp, a supercoiled plasmid, purified IstA and IstB together with nucleotides and Mg2+. Insertion of a single linear end-carrying fragment results in relaxation of the plasmid while concerted integration of a pair of ends (at the same site on opposite strands) results in cleavage of both plasmid strands and linearization.
Both IstA and IstB were required for concerted end integration and, moreover, while integration occurred using DNA end fragments with blunt ends, it appeared significantly more efficient if the end substrates carried a short 5’ overhang. Using an equimolar mixture of precleaved IRR and IRL, the linear insertion products were observed to carry one IRR and one IRL, two IRR or 2 IRL implying that the transposase shows no strong preference for either IS end.
Moreover, if only one end was used in the reaction, IRL alone was only two-fold less efficient that either IRR alone which was in turn similar to the activity of the IRR/IRL mix. Like some other transposons such as Mu and Tn7 [29][30], IS21 family members carry internal repeated sequences at each end (Fig. IS21.2; Fig. IS21.3): generally, 2-3 at the left (L1, L2 and L3) end and 2 at the right (R1 andR2). For both Mu and Tn7, these serve as binding sites for transposition enzymes [29][31][32].
Fluorescence anisotropy was used to examine the capacity of IstA to recognize different donor substrates. For this, the behavior of double strand precleaved DNA fragments including both R1 and R2, R1 alone or “random” sequences was analyzed in the presence of IstA tagged with MBP (Maltose Binding Protein) to improve solubility. IstA was found to exhibit stronger cooperative binding for the full-length R1-R2 DNA than for the R1 or random DNA sequences. In addition, size exclusion chromatography showed that IstA alone eluted as a monomer while in the presence of IS ends, IstA formed a stable, high molecular weight assembly. Negatively stained electron micrographs showed that these assemblies assumed an elongated shape with two-fold symmetry.
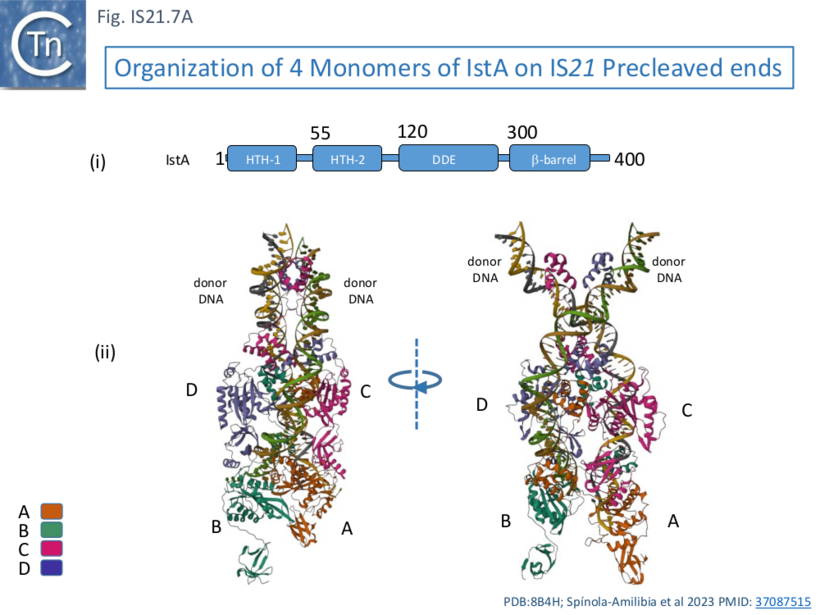
This behavior was similar whether or not the IS ends carried a 5’ overhang although the overhang was necessary for efficient strand transfer. Electron microscopy using biotin tagged DNA showed that the complex assemblies were PECs (Paired End Complexes) with two parallel DNA ends. Cryoelectron microscopy was used to probe the structure of the PECs. It was observed that resolution of assemblies built with either two left or two right ends was higher than those constructed with mixtures of left and right ends, presumably because of the heterogeneous nature of the assemblies (2 IRL, 2 IRR and IRL+IRR). Structures were resolved using IRR alone. This showed that IstA assembles as a tetramer on two IRs in which the monomers (A, B, C, and D; Fig. IS21.7A) are strongly interconnected.
IstA can be divided into a number of functional domains (Fig. IS21.7Ai): two helix-turn-helix domains presumably involved in sequence-specific binding to the IS ends (HTH-1 and HTH-2) located at the N-terminus, the catalytic DDE domain followed by a β-barrel domain at the C-terminus.
These four monomers in the transposition complex assembly clearly perform different roles: in the upper pair (C and D; Fig. IS21.7 Aii, Bii and iii), the DDE domains are too far from the DNA for catalysis and these probably serve as structural rather than catalytic monomers; while the lower pair (A and B) engage the ends in trans in which the HTH domains of one monomer bind to one end and its DDE domain is located in proximity to the tip of the other end correctly positioned for cleavage at the terminal 3’CA of the transferred strand, as is the case for a number of transposable elements [33][34][35][36]. This trans arrangement ensures that a functional transpososome is assembled before any chemistry can take place and helps to reduced the risk of abortive transposition events. Moreover, binding induces a base on the 5’, non-transferred strand to “flip” out, a characteristic in a number of transposition systems [37][33][38] as well as a number of other DNA transactions (see [37] for examples) which the authors suggest, may be involved in regulation of the chemistry.
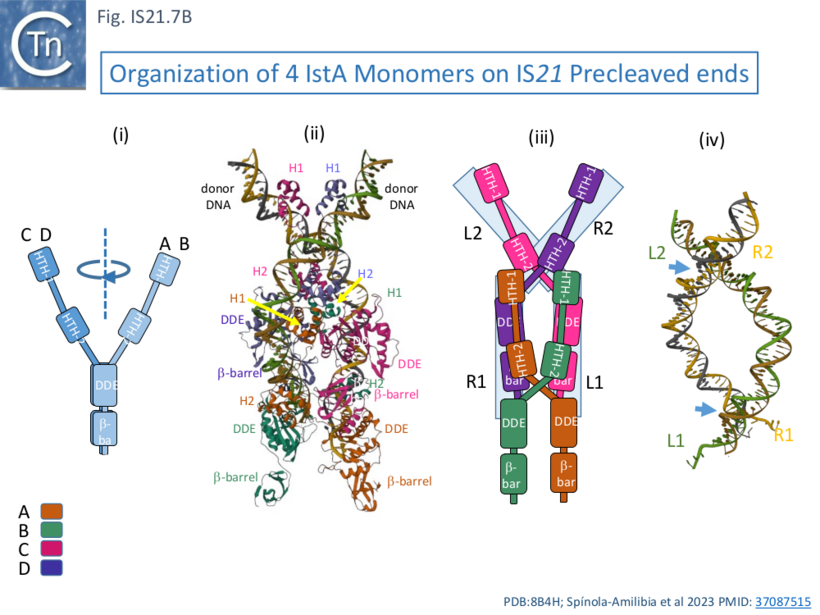
One important difference in the monomer pairs is that the HTH domains are rotated with respect to the DDE domains (Fig. IS21.7Bi). In binding, the tetrameric IstA traps two crossovers in the DNA ends (Fig. IS21.7Biv) which would result in introduction of two right-hand supercoils. In addition, as might be expected, the structure also revealed how IstA binding to the left IS end would repress transposase expression, a phenomenon observed with other IS.
Although these studies represent a considerable advance on out knowledge of IS21 family transposition, they do not address the interactions between IstA and the IstB ATPase in the overall process.
Bibliography
- ↑ 1.0 1.1 1.2 Riess G, Holloway BW, Pühler A . R68.45, a plasmid with chromosome mobilizing ability (Cma) carries a tandem duplication. - Genet Res: 1980 Aug, 36(1);99-109 [PubMed:6777257] [DOI]
- ↑ Willetts NS, Crowther C, Holloway BW . The insertion sequence IS21 of R68.45 and the molecular basis for mobilization of the bacterial chromosome. - Plasmid: 1981 Jul, 6(1);30-52 [PubMed:6269130] [DOI]
- ↑ Watson JM, Holloway BW . Chromosome mapping in Pseudomonas aeruginosa PAT. - J Bacteriol: 1978 Mar, 133(3);1113-25 [PubMed:417059] [DOI]
- ↑ 4.0 4.1 4.2 Reimmann C, Moore R, Little S, Savioz A, Willetts NS, Haas D . Genetic structure, function and regulation of the transposable element IS21. - Mol Gen Genet: 1989 Feb, 215(3);416-24 [PubMed:2540414] [DOI]
- ↑ Depicker A, De Block M, Inzé D, Van Montagu M, Schell J . IS-like element IS8 in RP4 plasmid and its involvement in cointegration. - Gene: 1980 Sep, 10(4);329-38 [PubMed:6253354] [DOI]
- ↑ Burland V, Shao Y, Perna NT, Plunkett G, Sofia HJ, Blattner FR . The complete DNA sequence and analysis of the large virulence plasmid of Escherichia coli O157:H7. - Nucleic Acids Res: 1998 Sep 15, 26(18);4196-204 [PubMed:9722640] [DOI]
- ↑ Hu P, Elliott J, McCready P, Skowronski E, Garnes J, Kobayashi A, Brubaker RR, Garcia E . Structural organization of virulence-associated plasmids of Yersinia pestis. - J Bacteriol: 1998 Oct, 180(19);5192-202 [PubMed:9748454] [DOI]
- ↑ Filippov AA, Oleinikov PV, Motin VL, Protsenko OA, Smirnov GB . Sequencing of two Yersinia pestis IS elements, IS285 and IS100. - Contrib Microbiol Immunol: 1995, 13;306-9 [PubMed:8833859]
- ↑ Buchrieser C, Brosch R, Bach S, Guiyoule A, Carniel E . The high-pathogenicity island of Yersinia pseudotuberculosis can be inserted into any of the three chromosomal asn tRNA genes. - Mol Microbiol: 1998 Dec, 30(5);965-78 [PubMed:9988474] [DOI]
- ↑ Swenson DL, Bukanov NO, Berg DE, Welch RA . Two pathogenicity islands in uropathogenic Escherichia coli J96: cosmid cloning and sample sequencing. - Infect Immun: 1996 Sep, 64(9);3736-43 [PubMed:8751923] [DOI]
- ↑ 11.0 11.1 Mahillon J, Chandler M . Insertion sequences. - Microbiol Mol Biol Rev: 1998 Sep, 62(3);725-74 [PubMed:9729608] [DOI]
- ↑ Soby S, Kirkpatrick B, Kosuge T . Characterization of an insertion sequence (IS53) located within IS51 on the iaa-containing plasmid of Pseudomonas syringae pv. savastanoi. - Plasmid: 1993 Mar, 29(2);135-41 [PubMed:8385786] [DOI]
- ↑ Matsutani S, Ohtsubo H, Maeda Y, Ohtsubo E . Isolation and characterization of IS elements repeated in the bacterial chromosome. - J Mol Biol: 1987 Aug 5, 196(3);445-55 [PubMed:2824781] [DOI]
- ↑ Koonin EV . DnaC protein contains a modified ATP-binding motif and belongs to a novel family of ATPases including also DnaA. - Nucleic Acids Res: 1992 Apr 25, 20(8);1997 [PubMed:1533715] [DOI]
- ↑ 15.0 15.1 Reimmann C, Haas D . Mode of replicon fusion mediated by the duplicated insertion sequence IS21 in Escherichia coli. - Genetics: 1987 Apr, 115(4);619-25 [PubMed:3034717] [DOI]
- ↑ Kiss J, Olasz F . Formation and transposition of the covalently closed IS30 circle: the relation between tandem dimers and monomeric circles. - Mol Microbiol: 1999 Oct, 34(1);37-52 [PubMed:10540284] [DOI]
- ↑ Turlan C, Ton-Hoang B, Chandler M . The role of tandem IS dimers in IS911 transposition. - Mol Microbiol: 2000 Mar, 35(6);1312-25 [PubMed:10760133] [DOI]
- ↑ 18.0 18.1 18.2 18.3 Reimmann C, Haas D . The istA gene of insertion sequence IS21 is essential for cleavage at the inner 3' ends of tandemly repeated IS21 elements in vitro. - EMBO J: 1990 Dec, 9(12);4055-63 [PubMed:2174355] [DOI]
- ↑ 19.0 19.1 Schmid S, Seitz T, Haas D . Cointegrase, a naturally occurring, truncated form of IS21 transposase, catalyzes replicon fusion rather than simple insertion of IS21. - J Mol Biol: 1998 Sep 25, 282(3);571-83 [PubMed:9737923] [DOI]
- ↑ 20.0 20.1 Berger B, Haas D . Transposase and cointegrase: specialized transposition proteins of the bacterial insertion sequence IS21 and related elements. - Cell Mol Life Sci: 2001 Mar, 58(3);403-19 [PubMed:11315188] [DOI]
- ↑ 21.0 21.1 Arias-Palomo E, Berger JM . An Atypical AAA+ ATPase Assembly Controls Efficient Transposition through DNA Remodeling and Transposase Recruitment. - Cell: 2015 Aug 13, 162(4);860-71 [PubMed:26276634] [DOI]
- ↑ 22.0 22.1 Spínola-Amilibia M, Araújo-Bazán L, de la Gándara Á, Berger JM, Arias-Palomo E . IS21 family transposase cleaved donor complex traps two right-handed superhelical crossings. - Nat Commun: 2023 Apr 22, 14(1);2335 [PubMed:37087515] [DOI]
- ↑ Peters JE . Tn7. - Microbiol Spectr: 2014 Oct, 2(5); [PubMed:26104363] [DOI]
- ↑ Harshey RM . Transposable Phage Mu. - Microbiol Spectr: 2014 Oct, 2(5); [PubMed:26104374] [DOI]
- ↑ Mizuno N, Dramićanin M, Mizuuchi M, Adam J, Wang Y, Han YW, Yang W, Steven AC, Mizuuchi K, Ramón-Maiques S . MuB is an AAA+ ATPase that forms helical filaments to control target selection for DNA transposition. - Proc Natl Acad Sci U S A: 2013 Jul 2, 110(27);E2441-50 [PubMed:23776210] [DOI]
- ↑ Xu K, He ZQ, Mao YM, Sheng RQ, Sheng ZJ . On two transposable elements from Bacillus stearothermophilus. - Plasmid: 1993 Jan, 29(1);1-9 [PubMed:8382825] [DOI]
- ↑ Goodarzi G, Im GJ, Brackmann K, Grandgenett D . Concerted integration of retrovirus-like DNA by human immunodeficiency virus type 1 integrase. - J Virol: 1995 Oct, 69(10);6090-7 [PubMed:7666512] [DOI]
- ↑ Li M, Craigie R . Processing of viral DNA ends channels the HIV-1 integration reaction to concerted integration. - J Biol Chem: 2005 Aug 12, 280(32);29334-9 [PubMed:15958388] [DOI]
- ↑ 29.0 29.1
Craigie R, Mizuuchi M, Mizuuchi K . Site-specific recognition of the bacteriophage Mu ends by the Mu A protein. - Cell: 1984 Dec, 39(2 Pt 1);387-94 [PubMed:6094016]
[DOI]
- ↑ Lichtenstein C, Brenner S . Unique insertion site of Tn7 in the E. coli chromosome. - Nature: 1982 Jun 17, 297(5867);601-3 [PubMed:6283361] [DOI]
- ↑ Tang Y, Lichtenstein C, Cotterill S . Purification and characterisation of the TnsB protein of Tn7: a transposition protein that binds to the ends of Tn7. - Nucleic Acids Res: 1991 Jun 25, 19(12);3395-402 [PubMed:1648205] [DOI]
- ↑
Arciszewska LK, McKown RL, Craig NL . Purification of TnsB, a transposition protein that binds to the ends of Tn7. - J Biol Chem: 1991 Nov 15, 266(32);21736-44 [PubMed:1657979]
- ↑ 33.0 33.1 Davies DR, Goryshin IY, Reznikoff WS, Rayment I . Three-dimensional structure of the Tn5 synaptic complex transposition intermediate. - Science: 2000 Jul 7, 289(5476);77-85 [PubMed:10884228] [DOI]
- ↑ Montaño SP, Pigli YZ, Rice PA . The μ transpososome structure sheds light on DDE recombinase evolution. - Nature: 2012 Nov 15, 491(7424);413-7 [PubMed:23135398] [DOI]
- ↑ Richardson JM, Colloms SD, Finnegan DJ, Walkinshaw MD . Molecular architecture of the Mos1 paired-end complex: the structural basis of DNA transposition in a eukaryote. - Cell: 2009 Sep 18, 138(6);1096-108 [PubMed:19766564] [DOI]
- ↑ Ghanim GE, Kellogg EH, Nogales E, Rio DC . Structure of a P element transposase-DNA complex reveals unusual DNA structures and GTP-DNA contacts. - Nat Struct Mol Biol: 2019 Nov, 26(11);1013-1022 [PubMed:31659330] [DOI]
- ↑ 37.0 37.1 Bischerour J, Chalmers R . Base flipping in tn10 transposition: an active flip and capture mechanism. - PLoS One: 2009 Jul 10, 4(7);e6201 [PubMed:19593448] [DOI]
- ↑ Grundy GJ, Hesse JE, Gellert M . Requirements for DNA hairpin formation by RAG1/2. - Proc Natl Acad Sci U S A: 2007 Feb 27, 104(9);3078-83 [PubMed:17307873] [DOI]