IS Families/IS630 family
Contents
Original Identification
IS630 was first identified in the Shigella sonnei genome[1]. Another transpositionally active IS630 family member which showed similarity to the Tc1 eukaryotic transposon family[2] has received most attention was subsequently identified in the cyanobacterium Synechocystis PCC6803 and called ISS1987[2] or ISTcSa and subsequently renamed ISY100[3].
Distribution
There are over 200 members from over 80 bacterial and archaeal genomes. IS630 itself has been used to cluster subspecies of Aeromonas salmonicida by high copy number IS630 restriction fragment length polymorphism (HCN-IS630-RFLP)[4].
Organization
Members are between 950 and 1250 bp in length with an average of 1100 bp (Fig. IS630.2). They have short terminal IRs (Fig. IS630.1) and generally include a single orf. However, in about nearly half of the members, the Tpase orf is distributed over two reading frames, suggesting that it may be produced as a fusion protein by frameshifting. These include IS895 and ISRm2011-2, identified several decades ago, which appear to contain two consecutive open reading frames[5].
Other member's families carry a single long reading frame. Three elements (IS870, ISAr1, and ISRf1) show more than 70% identity, two (IS1066 and ISRj1) show about 50% identity with each other and 40% with the other members, while two show less than 20% identity between themselves and with the other members. This is reflected in the relatively low similarity of their IRs (Fig. IS630.1).
It is interesting to note that the three most closely related on the basis of Tpase similarities (IS870, ISRf1, and ISAr1) appear not to carry translation termination codons for the Tpase gene. However, insertion into the specific target site, CTAG, with concomitant duplication (of either 2 or 4 base pairs) generates a TAG termination codon in phase with the Tpase gene. The influence of this arrangement on the transposition of the IS elements has yet to be determined.
Of the ISY100 copies in the Synechocystis PCC6803 genome, around 50% were found to include a single orf while the others included 2 smaller orfs which had been split by the presence of an additional A nucleotide in a stretch of 9 As[3]. While it is possible that these IS copies are inactive, it is also possible that a full-length transposase is expressed by frameshifting. Although the absence of the characteristic secondary structure signals associated with -1 programmed translational frameshifting suggests that this is unlikely, it remains possible that a transcriptional mechanism is operating. One member, ISRm2011-2 from Rhizobium meliloti, also carries a group II intron which appears to be active in vivo[6].
The IS630 family is related to the Tc1/mariner family of eukaryotic TE particularly at the level of the DDE signature[2] (Fig. IS630.1). There is also an N-terminal HTH motif[3] (Fig. IS630.3) whose function in binding ISY100 ends has been verified in vitro[7]. Moreover, IS630 and the Tc1/mariner families target similar sequences, have similar DR and transposition of both involves cleavage of two nucleotides inside the 5’ ends[7][8].
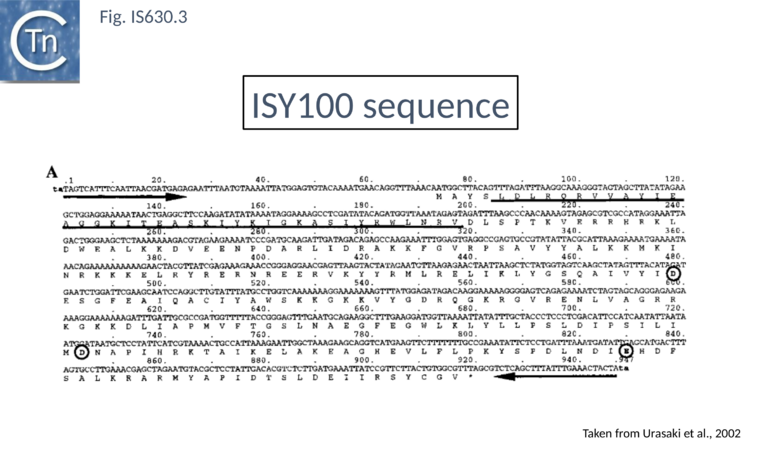
Analysis of the Streptococcus pneumoniae genome revealed several hundred copies of short imperfect palindromic sequences (RUPs) whose ends show strong homology to those of a full length putative IS630-related element also present in the genome[9]. Comparison of empty and full sites from different S. pneumoniae strains indicated that, like most full-length members of the family, the RUPs are flanked by a TA dinucleotide target repeat. Although transposition of RUP sequences has yet to be demonstrated, they are structurally similar to the IS231-related MIC231 elements in B. thuringiensis (see "IS4 family") and to several eukaryote systems where many truncated copies of an element may exist together with a full length functional copy that is capable of complementing the non-autonomous copies to drive their transposition. Other IS630-based MITES have been identified in Escherichia coli, Photorhabdus luminescens[10] and Yersinia pestis[11].
Insertion Specificity
Family members show high target specificity inserting into and duplicating a TA dinucleotide, with a preference for the sequence 5’-NTAN-3’[12]. Since the cleavages of the non-transferred strand occur 2 nts within the 5’ end of the IS, repair of the donor molecule after excision of the IS can result in a 2 bp scar at the excision site.
Detailed studies concerning the target DNA sequence have been carried out in the case of IS630[13]. The target sequence was determined before and after insertion, and the results suggested that insertion generated a duplication of an invariant target TA dinucleotide. That this dinucleotide does not form an integral part of the IS was investigated by site-specific mutagenesis of the transposon donor to eliminate the terminal TA. Transposition of the IS from the mutated donor molecule resulted in insertions which all exhibited a flanking TA direct repeat. This clearly demonstrates that insertion results in the duplication of the central TA dinucleotide[13]. Further analysis demonstrated that IS630 exhibits a strong preference for a 5'-CTAG-3' target sequence[12]. Point mutation of the CTAG target sites reduced or eliminated their attractiveness as insertion hotspots. The two preferred insertion sites were identified in plasmid ColE1 corresponded to TA sequences in the inverted repeats of a 13-base-pair stem region of the [rho]-dependent transcription terminator. IS630 is flanked by TA, and in vitro mutagenesis of the flanking TA did not affect further transposition activity, the ability to insert preferentially into the TA within the 13-base-pair inverted repeat or to duplicate its target sequence[12][13].
All known insertions of these elements are consistent with a TA duplication. In two insertions of the Agrobacterium vitis element IS870 it was possible to determine the target sequence prior to insertion. This was found to carry a single CTAG copy, an observation that remains consistent with a simple TA dinucleotide target duplication. We assume that all members of this family generate an identical (TA) target duplication, and the tips of the elements have therefore been defined accordingly in ISfinder.
Mechanism
In vivo studies [3] demonstrated that, when supplied with transposase, the IS630 family member, ISY100 (ISTcSa) first identified in Synechocystis sp. PCC6803[2] generates linear forms which terminate exactly at the 3’ ends but which lack 2 nucleotides at the 5’ ends resulting in a 2 base overhang. Both in vivo [3] and in vitro using circular plasmid DNA[7]. This is also a characteristic of transposition of the eukaryote Tc1 transposon[14]. Although IS circles had been observed, as for Tc1 these are thought to be dead-end products and not transposition intermediates[3].
In the case of IS630 itself, while direct insertions can be observed at reasonable frequencies, no cointegrates could be detected[13].
IS630 transposition has been addressed in vitro using ISY100[7]. The Tpase was shown to specifically bind ISY100 IR using an N-terminal domain containing two potential HTH motifs. It is the only protein required for ISY100 excision and integration and introduces double-strand breaks on mini-ISY100 on a supercoiled DNA substrate. Tc1/mariner element transposition has also been extensively studied in vitro[8] and a Tpase structural model is available [e.g. [15][16]]. IS630 Tpase cleaves exactly at the 3’ (transferred strand) IS ends and two nucleotides inside the 5’ (non-transferred strand) ends. Cleavage is less precise on linear substrates. Both single-end and, less frequently, double-end insertion occur in vitro in a TA-target-specific manner[17]. Transposition does not involve a hairpin intermediate.
The related eukaryote Tc1 element is known to transpose by a cut-and-paste mechanism and leaves a “footprint” (additional bases in the original donor site) on excision (Fig. IS630.4). When purified vector backbone produced by transposase mediated cleavage of ISY100 in vitro was circularised In vitro, gel- produced by transposase-mediated cleavage of pISY100 -kan was efficiently circularized with T4 DNA ligase the predicted TATATA (i.e. a footprint) junction sequence was recovered after transformation into E. coli.
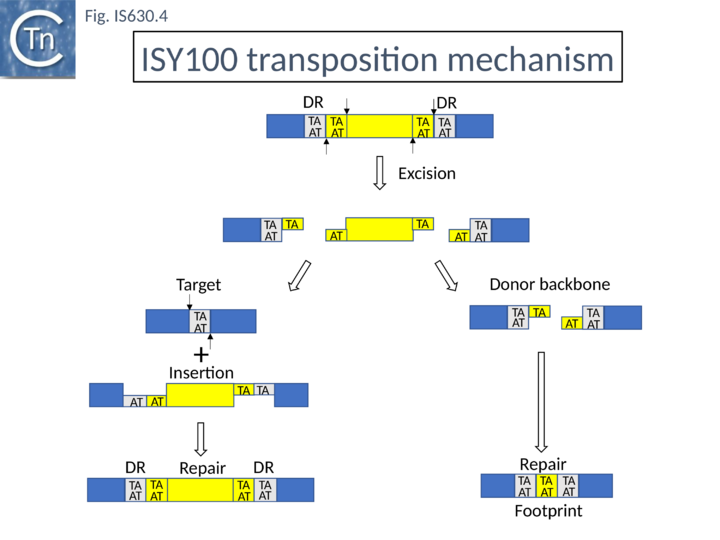
It has also been demonstrated that fusion of a zinc-finger DNA-binding domain of Zif268 to the ISY100 transposase C-terminus targets integration into TA dinucleotides positioned 6-17 bp to one side of a Zif268 binding site. The targeting specificity can be changed with Zif268 variants which recognize other sequences[17].
Bibliography
- ↑ Matsutani S, Ohtsubo H, Maeda Y, Ohtsubo E . Isolation and characterization of IS elements repeated in the bacterial chromosome. - J Mol Biol: 1987 Aug 5, 196(3);445-55 [PubMed:2824781] [DOI]
- ↑ 2.0 2.1 2.2 2.3 Cassier-Chauvat C, Poncelet M, Chauvat F . Three insertion sequences from the cyanobacterium Synechocystis PCC6803 support the occurrence of horizontal DNA transfer among bacteria. - Gene: 1997 Aug 22, 195(2);257-66 [PubMed:9305771] [DOI]
- ↑ 3.0 3.1 3.2 3.3 3.4 3.5 Urasaki A, Sekine Y, Ohtsubo E . Transposition of cyanobacterium insertion element ISY100 in Escherichia coli. - J Bacteriol: 2002 Sep, 184(18);5104-12 [PubMed:12193627] [DOI]
- ↑ Studer N, Frey J, Vanden Bergh P . Clustering subspecies of Aeromonas salmonicida using IS630 typing. - BMC Microbiol: 2013 Feb 13, 13;36 [PubMed:23406017] [DOI]
- ↑ Alam J, Vrba JM, Cai Y, Martin JA, Weislo LJ, Curtis SE . Characterization of the IS895 family of insertion sequences from the cyanobacterium Anabaena sp. strain PCC 7120. - J Bacteriol: 1991 Sep, 173(18);5778-83 [PubMed:1653219] [DOI]
- ↑ Martínez-Abarca F, Zekri S, Toro N . Characterization and splicing in vivo of a Sinorhizobium meliloti group II intron associated with particular insertion sequences of the IS630-Tc1/IS3 retroposon superfamily. - Mol Microbiol: 1998 Jun, 28(6);1295-306 [PubMed:9680217] [DOI]
- ↑ 7.0 7.1 7.2 7.3 Feng X, Colloms SD . In vitro transposition of ISY100, a bacterial insertion sequence belonging to the Tc1/mariner family. - Mol Microbiol: 2007 Sep, 65(6);1432-43 [PubMed:17680987] [DOI]
- ↑ 8.0 8.1 Plasterk RH . The Tc1/mariner transposon family. - Curr Top Microbiol Immunol: 1996, 204;125-43 [PubMed:8556864] [DOI]
- ↑ Oggioni MR, Claverys JP . Repeated extragenic sequences in prokaryotic genomes: a proposal for the origin and dynamics of the RUP element in Streptococcus pneumoniae. - Microbiology (Reading): 1999 Oct, 145 ( Pt 10);2647-53 [PubMed:10537186] [DOI]
- ↑ Duchaud E, Rusniok C, Frangeul L, Buchrieser C, Givaudan A, Taourit S, Bocs S, Boursaux-Eude C, Chandler M, Charles JF, Dassa E, Derose R, Derzelle S, Freyssinet G, Gaudriault S, Médigue C, Lanois A, Powell K, Siguier P, Vincent R, Wingate V, Zouine M, Glaser P, Boemare N, Danchin A, Kunst F . The genome sequence of the entomopathogenic bacterium Photorhabdus luminescens. - Nat Biotechnol: 2003 Nov, 21(11);1307-13 [PubMed:14528314] [DOI]
- ↑ De Gregorio E, Silvestro G, Venditti R, Carlomagno MS, Di Nocera PP . Structural organization and functional properties of miniature DNA insertion sequences in yersiniae. - J Bacteriol: 2006 Nov, 188(22);7876-84 [PubMed:16963573] [DOI]
- ↑ 12.0 12.1 12.2 Tenzen T, Ohtsubo E . Preferential transposition of an IS630-associated composite transposon to TA in the 5'-CTAG-3' sequence. - J Bacteriol: 1991 Oct, 173(19);6207-12 [PubMed:1655702] [DOI]
- ↑ 13.0 13.1 13.2 13.3 Tenzen T, Matsutani S, Ohtsubo E . Site-specific transposition of insertion sequence IS630. - J Bacteriol: 1990 Jul, 172(7);3830-6 [PubMed:2163390] [DOI]
- ↑ Plasterk RH, Izsvák Z, Ivics Z . Resident aliens: the Tc1/mariner superfamily of transposable elements. - Trends Genet: 1999 Aug, 15(8);326-32 [PubMed:10431195] [DOI]
- ↑ Dawson A, Finnegan DJ . Excision of the Drosophila mariner transposon Mos1. Comparison with bacterial transposition and V(D)J recombination. - Mol Cell: 2003 Jan, 11(1);225-35 [PubMed:12535535] [DOI]
- ↑ Richardson JM, Dawson A, O'Hagan N, Taylor P, Finnegan DJ, Walkinshaw MD . Mechanism of Mos1 transposition: insights from structural analysis. - EMBO J: 2006 Mar 22, 25(6);1324-34 [PubMed:16511570] [DOI]
- ↑ 17.0 17.1 Feng X, Bednarz AL, Colloms SD . Precise targeted integration by a chimaeric transposase zinc-finger fusion protein. - Nucleic Acids Res: 2010 Mar, 38(4);1204-16 [PubMed:19965773] [DOI]